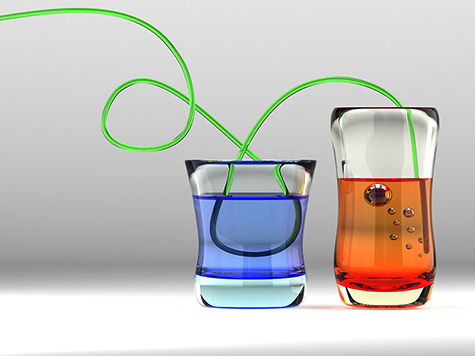
Only recently has it become possible to accurately “see” the structure of a liquid. Using X-rays and a high-tech apparatus that holds liquids without a container,Kenneth Kelton, PhD, the Arthur Holly Compton Professor in Arts & Sciences at Washington University in St. Louis, was able to compare the behavior of glass-forming liquids as they approach the glass transition.
The results, published in the August 6 issue of Nature Communications, are the strongest demonstration yet that bulk properties of glass-forming liquids, such as viscosity, are linked to microscopic ones, such as structure.
Although people have known how to make glass for thousands of years, the glassy state and the glass transition are still not fully understood. The method used to make most glasses provides a hint of the problem, Kelton said.
A liquid must first be cooled below its freezing temperature (supercooled) without crystallizing. As the temperature of the supercooled liquid drops further, the liquid becomes more and more viscous. Eventually it reaches a point where its molecules or atoms can’t move fast enough to accommodate changes in temperature, and portions of the liquid successively jam, or lock in place.
The transition from a liquid to a glass is not a phase transition, like the familiar conversion of water to ice. At the freezing point, water and ice are both states in thermodynamic equilibrium, meaning everything within them is in balance and nothing is driving them to change. Glass, on the other hand, is not in equilibrium at any temperature.
Despite years of study, the process of glass formation still puzzles scientists. For the most part they have only been able to measure bulk properties of glass-forming liquids, such as viscosity and specific heat, and the interpretations they came up with depended in part on the measurements they took. But they were aware that these properties probably reflected changes in the liquid’s structure at an atomic level.
Understanding the glass transition is important, Kelton said, because glasses are far more common than people realize. “One day I noticed that some of my nuts-and-bolts papers on the crystallization of glasses were much more highly cited than I would have expected,” he said. “It turned out they were being cited by people in the pharmaceutical industry.”
Pharmaceutical companies have been developing “amorphous” (glassy) drugs for a variety of reasons. But one reason that amorphous drugs generally dissolve better in the body, so that lower doses are more effective.
But that’s just one of many hidden uses for glass science.
Fragile and strong liquids
Kelton chose to study a property called liquid fragility,’ which appears to play a role in glass formation. The term ‘fragility’ was first coined in 1995 by Austen Angell, now a professor of chemistry at Arizona State University, who is known for his research on the physics of glasses and glass-forming liquids. Angell felt that a new term was needed to capture dramatic differences in the way a liquid’s viscosity increases as it approaches the glass transition.
The viscosities of some liquids change gradually and smoothly as they approach this transition. But as others are cooled, the viscosities change very little at first, but then take off like a rocket as the transition approaches.
Angell could only measure viscosity, but he called the first type of liquid “strong” and the second type “fragile” because he suspected a structural difference underlay the differences that he saw.
“It’s easier to explain what he meant in terms of the transition from a glass to a liquid rather than the other way around,” Kelton said. “Suppose a glass is heated through the glass transition temperature. If it’s a strong system, it ‘remembers’ the structure it had as a glass—which is more ordered than in a liquid—and that tells you that the structure does not change much through the transition. In contrast, a fragile system quickly ‘forgets’ its glass structure, which tells you that its structure changes a lot through the transition.
“That ‘s how Angell viewed it,” Kelton continued, “but it had never been experimentally shown. People argued that the change in viscosity had to be related to the structure—through several intermediate concepts, some of which are not well defined. What we did was hop over these intermediate steps to show directly that fragility was related to structure.”
Putting glass under the “microscope”
Kelton was able to look at structure because his team has built a new apparatus, the Washington University-Beamline Electrostatic Levitator, or WU-BESL, which was specifically designed to provide a kind of “microscope” to study the atomic structure of liquids, much like a traditional microscope can look into the body of a cell.
In a sense the WU-BESL isn’t all that different from the familiar light microscope. But instead of being clipped on a stage, the supercooled sample is levitated in a vacuum to avoid contact with a container or even a floating speck of dust, which could make it suddenly crystallize.
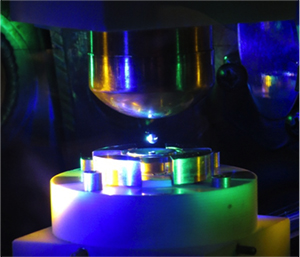
And instead of probing the sample with visible light, which has wavelengths too long to resolve atoms, it probes them with high intensity X-rays. During an experiment, the WU-BESL is carried to Argonne National Laboratory outside of Chicago and installed at the Advanced Photon Source, a particle accelerator that produces an intense beam of X-ray photons.
Once levitated, the sample is melted with a high-power laser and allowed to cool. As its temperature decreases toward the glass transition, the sample is exposed to the X-ray photons and detectors measure the intensity of scattered photons as a function of the scattering angle.
By analyzing the scattered data, the scientists obtain a plot of “the structure factor,“ an oscillating line with peaks of rapidly diminishing height that contains information on atom locations. Kelton and his colleagues focus on the first of these peaks, which corresponds most directly with the change in the liquid’s average structure.
To test Angell’s idea, the scientists heated and then cooled many samples of metallic glass-forming liquids, some considered strong and the others fragile, in the WU-BESL. The strong liquid’s structure factor evolved gradually from that of a liquid to that of a glass. But the evolution of the fragile liquid’s structure factor accelerated abruptly as it approached the glass transition.
It was just as Angell had suspected. The rate of atomic ordering in the liquid near the transition temperature determines whether a liquid is fragile or strong.
These results help us understand some fundamental physics, but Kelton also points out that they have a practical importance, providing glass manufacturers with a new way to search for good glass formers. Strong liquids seem to be good glass formers that “want” to form glasses and ignore inducements to crystallize. Fragile liquids are generally bad glass formers that can be coaxed to make glass only by using extreme manufacturing techniques.
Since 2010, Apple Inc. has had an exclusive rights agreement with Liquidmetal Technologies, a company that make metallic glasses, Kelton said. In May 2014, Apple was granted a patent for “printing” metallic glass bezels for smartphones, which will be stronger than plastic ones, but more flexible than metal ones.
“By learning how liquid structure is related to the formation of liquid glasses,” Kelton said, “we open the door a bit wider to the invention of new metallic glasses for novel applications.”