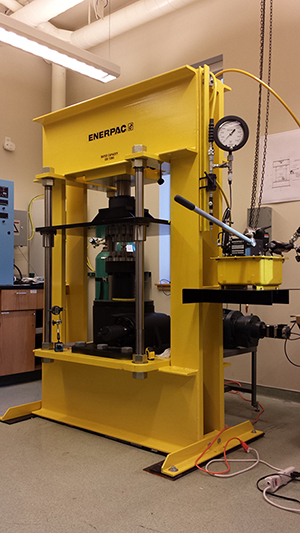
lab. The yellow frame is a commercial hydraulic press rated at 100 tons
and the black cylindrical object poking out to the bottom right is the
actuator that twists the bottom anvil of the press. (Credit: SKEMER LAB)
“I have always been interested in the origins of plate tectonics,” said Philip Skemer, PhD, assistant professor in the Department of Earth
and Planetary Sciences in Arts & Sciences at Washington University in
St. Louis.
“Plate tectonics is directly or indirectly responsible for virtually every geological phenomenon we experience at Earth’s surface.”
“But when I got to graduate school I realized that many of the questions I was asking about plate tectonics couldn’t be answered using a rock hammer or a computer. To get to the heart of the matter, I really needed to do laboratory experiments.”
But as he quickly learned, there were no instruments that could recreate on the surface the conditions in Earth’s mantle. So with the help of a National Science Foundation grant, he built his own machine, which he calls the Large Volume Torsion apparatus.
The problem
Rocks on the surface of Earth are brittle and fracture if you hit them with a hammer. But deep below the surface, where it is much hotter and the pressure is much greater, they are able to deform without breaking. They creep, or flow, behaving more like fluids than rigid solids.
The flow manifests itself at the surface as the ponderous movement of large rafts of rock that make up the continents and the ocean floor. The flowing mantle rocks drag on these tectonic plates, pulling and pushing them this way and that.
“The viscosity of rocks is critical to understanding plate tectonics,” Skemer said. “At sufficiently high temperatures and pressures, rocks, like other fluids, have viscoity. But the viscosity of rocks in the upper mantle might be 1019 or 1020 pascal-seconds, whereas the viscosity of water is about 10-3 pascal-seconds. The difference is enormous; more than 20 orders of magnitude.
Scientists can estimate the viscosity of mantle materials under a variety of conditions by conducting experiments. But to derive viscosity without extrapolating dangerously beyond experimental data, they have to be able to expose rocks to a wide range of deformation conditions.
“For a long time, people were doing experiments with devices that compress a cylindrical rock sample,” Skemer said. “If I push on a cylinder, I might be able to shorten it by 50 percent, that is, strain it by 50 percent. But 50 percent is nothing for the Earth.
“If I go out in the field I can quite easily find rocks that deformed to strains of 2,000 percent or even larger,” he said. Many critical deformation processes do not occur until very large strains are achieved.
The new rig
To reach those levels of strain, Skemer has built a rock-deformation apparatus that twists samples as well as pressing on them. “If you twist a rock cylinder, you can twist it forever,” he said. “There’s no geometric limit to how much you can deform it.”
“I couldn’t just go out and buy the instrument I wanted,” Skemer said. “There are no commerically available rock-deformation instruments because the academic discipline is so small.
“Fortunately, there’s this whole world of equipment out there that will allow you to construct an apparatus that can do just about anything you want to do,” said Skemer, who admits to reading industrial supply catalogs for fun.
His new rig traps a rock sample between tungsten carbide anvils about a quarter inch in diameter within a 100-ton hydraulic press. Once it is under pressure a screw actuator, originally designed for tasks such as lifting drawbridges, twists it from below. Skemer has geared the actuator down by about 500,000:1, so the torque can be applied slowly.
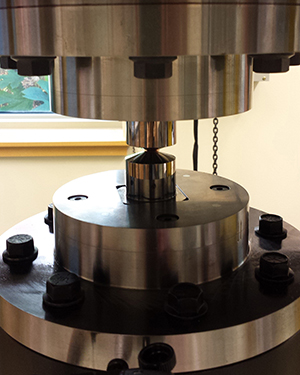
“Six giga-pascals (GPa) is my target pressure,” Skemer said. “That’s about 870,000 pounds per square inch. Put another way that’s 435 tons of force, about the weight of a loaded 747, pressing down on an area the size of a postage stamp. The center of the Earth is about 360 GPa,” he said, “but 6 GPa takes you 250 kilometers down, to the base of the tectonic plates.”
The sample will simultaneously be heated to temperatures as high as 1300 degrees Celsius (2,500 degrees Fahrenheit).
What about seismic data?
One of the many things Skemer wants to study with his new machine is how rocks under pressure develop what is called a lattice-preferred orientation, a key parameter in the interpretation of seismic data, which are used to image the interior of Earth much as CTs are used to image the interior of the human body.
Seismic waves travel faster in some directions than in others, a property called anisotropy. This happens because some mantle rocks have a preferred lattice orientation, or a preferred arrangement of crystalline grains and the bonds between their constituent atoms. A seismic wave will travel faster parallel to stiffer bonds and more slowly parallel to weaker bonds.
“Most of what we know about the patterns and direction of mantle flow comes from seismic data,” Skemer said. “The problem is that assumptions must be made about the relationships among flow, seismic anisotropy and lattice-preferred orientation in order to interpret the seismic data.
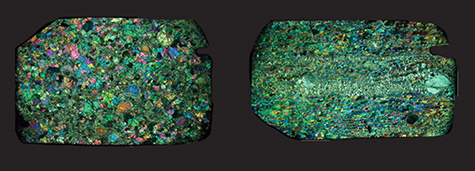
“How olivine, the dominant mantle mineral, goes from a random distribution of lattices to an organized one is not well understood,” said Skemer. “And if you don’t understand that, you can’t go in the other direction and use seismic anisotropy to infer the flow patterns in the mantle.
“As an experimentalist, I’m doing the forward problem, and seismologists are doing the inverse problem. They’re trying to go from anisotropy to flow, and I’m trying to understand how the anisotropy arises in the first place. They are complementary approaches; we cannot do one without the other.
“For decades, seismologists have been using simple models that assume the fastest direction for seismic waves is parallel to the direction of flow,” he said, “and I don’t think that assumption is always correct. It rests heavily on a small number of data that I think give an incomplete picture of what’s going on down there.
Anyone who reads the history of science can think of many instances where something true on the face of it turned out to be false when it was tested by experiment. That’s what drives him to roll up his sleeves and do experiments with the rock itself instead of by simulation, that is mathematically, and all on paper.