Cells are the basic units of structure and function in all forms of life on Earth, from humans and animals to yeasts and bacteria. But a cell itself is made up of even smaller working pieces called organelles. Understanding how a cell commits resources to building new parts — and eventually divides into two cells — is the focus of a new grant for physicist Shankar Mukherji of Washington University in St. Louis.
Mukherji was awarded a five-year $1.97 million grant for his project, “The design principles of the eukaryotic cell: uncovering the coordination of systems-level organelle dynamics, metabolism and growth.” The research project is funded by the National Institutes of Health (NIH).
“Our first order of business is to map out the relationship between the organelle biogenesis program within the cell as it relates to the growth and size of cells,” said Mukherji, assistant professor of physics in Arts & Sciences.
“We have some preliminary results on this,” he said. “For example, we have seen that there are collections of organelles that seem to work together to promote different growth rates. Now we are analyzing experimental results using data science techniques to look at these complicated data sets and figure out the relationships.
“Then we’ll be able to go in and actually perturb the biogenesis programs of individual organelles that you see pop up in the statistics and see how that affects the size and growth regulation of the cells.”
While some of his Crow Hall colleagues design and build X-ray detectors or measure electrical charges across atomically thin materials, Mukherji is a biophysicist — and he needs to keep his research subjects alive.
The cells he studies are the same kind of ordinary yeasts that bakers and brewers use to make bread, beer or wine. Other biophysics research at Washington University is focused on cellular motion, information processing in the brain and on the theoretical physics of ecology and evolution.
Because Mukherji’s modeling work depends on precise but systems-level measurements of living cells, researchers in his laboratory engineered a strain of yeast whose major organelles are fluorescently labeled, a yeast which they jokingly refer to as “rainbow yeast.”
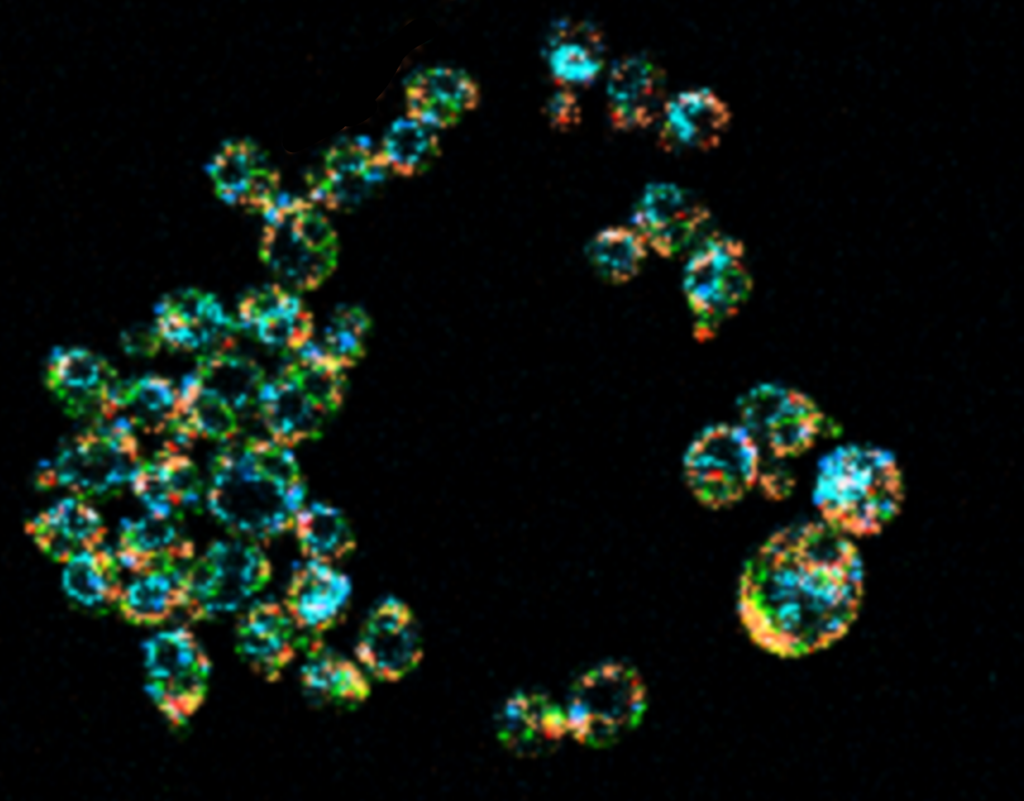
Imaging these cells, however, comes with challenges.
“Historically, when people tried to look at many things at once inside the cell, it was very hard to tell them apart — because the palette of colors we have to work with when we label structures inside cells is very limited and bleed into each other,” he said.
To overcome this problem, researchers in Mukherji’s group are combining previously developed optical tools and machine learning algorithms in a new way that allows them to apply hyperspectral imaging techniques to their rainbow yeast for long-term, live cell imaging.
This approach allows them to take pictures of multiple components of individual cells in action simultaneously.
Ultimately, Mukherji will use the data he collects to develop and test a mathematical model that quantitatively captures the connection between gene expression, metabolism and cellular growth dynamics as mediated by the cell’s organelle makeup. Such “whole cell models” that predict how the diverse scales of biological organization interact to produce physiological function is considered among the holy grails of quantitative biology and biophysics.
His new grant will help to uncover potential mechanisms that underlie how cells develop normally into different cell types. Mukherji’s findings also will be useful in the context of disease, such as when studying cancer or diabetes.
Diseased cells tend to end up looking and working differently than healthy cells. By understanding how cells remodel their organelle composition to handle altered metabolic profiles, Mukherji could potentially point toward new targets for therapies.
“For a cell to function properly, it must carry out a bunch of chemical reactions known as metabolism,” said Gary Patti, the Michael and Tana Powell Professor of Chemistry, who has worked with Mukherji on previous projects. “If you just mixed everything together in a giant pot, however, it wouldn’t work. Each chemical reaction requires specialized conditions, some of which are quite different.”
Cells solve this problem by creating tiny little compartments with unique chemical environments.
“Remarkably, we know very little about how the chemistry and physics are coordinated between these subcellular compartments, largely because approaches to study biochemical interactions at such a small scale have been limited,” Patti said. “Shankar has established techniques that will provide unprecedented resolution. The potential for discovery is extraordinary.”
In addition to teaching introductory physics and working with other scientists like Patti across Arts & Sciences, Mukherji is a member of the interdisciplinary Center for Science and Engineering of Living Systems. Mukherji also holds a joint appointment in cell biology and physiology at the School of Medicine, where he works closely with David W. Piston.
“Physicists made their name by combining theory and quantitative experiments to understand the organization of matter, from subatomic to astrophysical scales,” Mukherji said.
“Trying to understand how the eukaryotic cell organizes itself offers us the opportunity to work in that grand tradition, but in a very different conceptual context: one that is shaped by biological function and evolution,” he said. “We hope our work illustrates both the utility of tools such as mathematical models in biology but also the depth of the puzzles awaiting the next generation of physicists studying living systems.”