Membraneless organelles are tiny droplets inside a single cell, thought to regulate everything from division, to movement, to its very destruction. A better understanding of these mysterious structures could hold the key to unlocking a whole host of medical conditions, including developmental disorders, childhood cancers and age-related diseases.
New research from the School of Engineering & Applied Science at Washington University in St. Louis, published in the journal eLife, uncovers the principles underlying the formation and organization of membraneless organelles.
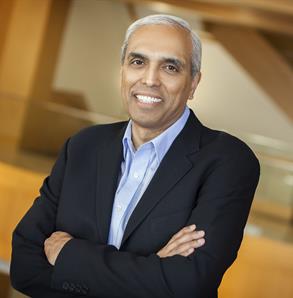
“If our theory and modeling are correct, we ought to be able to design these organelles in the way we want to,” said Rohit Pappu, the Edwin H. Murty Professor of Engineering in the Department of Biomedical Engineering.
Protein molecules that drive the formation of membraneless organelles are like pearl necklaces. They consist of multiple sticky domains (the pearls) connected by flexible linkers (the necklace). These so-called multivalent proteins come together to form networks that are held together by physical crosslinks among the sticky domains. The number of domains within a molecule contributes to the number of crosslinks that are realizable.
However, the phenomenon of crosslinking explains only half the story of how membraneless organelles form. These organelles are also known as “condensates,” because multivalent proteins actually condense to form droplets, and this cannot be explained by crosslinking alone.
Pappu’s team wondered if the ability to form spherical droplets is determined by the flexible linkers that tether domains to one another. Working with Michael Rosen of the University of Texas Southwestern Medical Center, Pappu and his colleagues, postdoctoral fellows Tyler Harmon and Alex Holehouse, used computer simulations and polymer physics theory to show that the sequence-specific physical properties of linkers directly determine whether multivalent proteins form gels within spherical condensates or gels without forming droplets.
“We were able to identify the sequence features that promote the formation of spherical gels, which is what these membraneless organelles really are,” Pappu said. “We should therefore be able to design droplets with bespoke material properties, and start to make sense of how and why specific types of proteins drive droplet formation and how these droplets contribute to cellular functions.
“Being able to mimic and design naturally occurring structures is the dream of biophysical engineering, and we are excited about what lies in store.”
Read more about the published research in eLife.
The study was funded by grants from: National Institutes of Health (RO1-GM56322); National Science Foundation (MCB1614766), Howard Hughes Medical Institute and St. Jude Children’s Research Hospital.