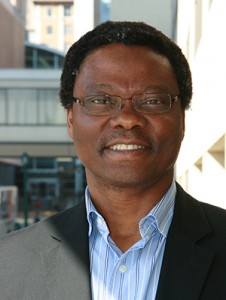
Light long has been used to treat cancer. But phototherapy is only effective where light easily can reach, limiting its use to cancers of the skin and in areas accessible with an endoscope, such as the gastrointestinal tract.
Using a mouse model of cancer, researchers at Washington University School of Medicine in St. Louis have devised a way to apply light-based therapy to deep tissues never before accessible. Instead of shining an outside light, they delivered light directly to tumor cells, along with a photosensitive source of free radicals that can be activated by the light to destroy cancer. And they accomplished this using materials already approved for use in cancer patients.
The study appears March 9 in the journal Nature Nanotechnology.
“Phototherapy works very well and has few side effects, but it can’t be used for deeply embedded or metastatic tumors,” said senior author Samuel Achilefu, PhD, professor of radiology and of biomedical engineering at Washington University. “In general, shining a light on photosensitive materials generates free radicals that are very toxic and induce cell death. But the technique has only worked well when light and oxygen can get there. The need for oxygen and the shallow penetration of light in tissue have limited advances in this area for decades.”
The light source the researchers harnessed relies on a phenomenon called Cerenkov radiation, identified in the 1930s by Pavel Cerenkov, who later won the Nobel Prize in Physics for the discovery. Cerenkov radiation is responsible for the characteristic blue glow of underwater nuclear reactors. It also is produced during positron emission tomography (PET) scans that doctors use to diagnose cancer.
Achilefu and first author Nalinikanth Kotagiri, MD, PhD, a postdoctoral researcher, focused on a widely used imaging strategy called FDG-PET. With this technique, patients undergo a PET scan after receiving an intravenous dose of radiolabeled sugar molecules called fluorodeoxyglucose (FDG). Many tumors take up the sugar to support their rapid growth, and the attached radioactive fluorine makes those tumors light up on a PET scan, no matter where they are in the body.
The researchers hypothesized that the radioactive fluorine also would produce enough Cerenkov radiation to activate a photosensitizing agent if it could also be delivered to the same location.
In this way, FDG could serve two purposes, continuing its role as an imaging agent and adding the new job of providing light for phototherapy, according to Kotagiri.
“FDG is one of the most widely used imaging agents in the world,” Achilefu said. “That’s the beauty of this treatment paradigm. It’s used in hospitals today to find primary and metastatic cancer. So with FDG as our light source, we needed to find a material that becomes toxic when exposed to the light it produces.”
After looking at a number of options, the researchers focused on nanoparticles made of titanium dioxide, a mineral with wide applications in medicine and industry including in hip implants, sunscreen, toothpaste and food additives. When exposed to light, titanium dioxide produces free radicals without requiring oxygen for the reaction. To see if they could increase the potency of the nanoparticles, the investigators also added a drug called titanocene to the nanomaterial’s surface.
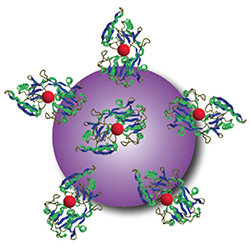
“Titanocene has been approved for investigational use in people,” Achilefu said. “It went all the way to Phase 2 clinical trials as a chemotherapy agent. It was found to be safe, but it didn’t work that well compared with a placebo. Still, it’s also known to interact with low-intensity light and break into free radicals. We decided to see if we could teach it to do its job differently — to act as a phototherapeutic drug instead of a chemotherapeutic drug.”
To help the nanoparticles home in on tumors in mice, the researchers also coated the particles with a protein called transferrin that binds to iron in the blood. Like sugar, many tumors rely on iron to grow. Achilefu pointed out that this iron-binding protein is simply one example of a way to target the photosensitive materials to cancer cells.
The researchers tested different formulations of the nanoparticles and cancer drug combined with the FDG light source in mice with human lung tumors and fibrosarcoma, a tumor of the connective tissue. Comparing these mice with untreated mice, they tested the following combinations: FDG plus tumor-seeking nanoparticles alone (no cancer drug), FDG plus tumor-seeking cancer drug alone (no nanoparticles), and FDG plus tumor-seeking nanoparticles carrying the cancer drug.
When injected into the bloodstream with FDG, the tumor-seeking nanoparticles that carried the cancer drug had the most significant effect. Fifteen days after treatment, tumors in treated mice were eight times smaller than those in untreated mice.
Mice that received FDG plus tumor-seeking nanoparticles alone survived about 30 days compared to an average of 15 days for untreated mice. They also found about the same 30-day survival for mice that received FDG plus just the tumor-seeking cancer drug — without the nanoparticles. Survival increased to 50 days for mice receiving all three components: FDG plus the tumor-seeking nanoparticles carrying the cancer drug.
“Exposed to the light source, the titanium dioxide nanoparticles alone can kill cancer,” Achilefu said. “But adding the drug appears to enhance the therapeutic outcome. The two together produce different kinds of free radicals that overwhelm tumor cells. Our formulation also uses doses of the drug that are much lower than would be administered for chemotherapy.”
Kotagiri added that toxic side effects should be minimal. Both the light and the photosensitive material are targeted to the tumor, and the material is not toxic unless activated by the light source, which should occur only at the tumor site.
Achilefu and Kotagiri are planning a small clinical trial in people to evaluate the readily available components of this strategy, beginning with FDG combined with the investigational cancer drug.