A chemist at Washington University in St. Louis is making molecules the new-fashioned way — selectively harnessing thousands of minuscule electrodes on a tiny computer chip that do chemical reactions and yield molecules that bind to receptor sites. Kevin Moeller, Ph.D., Washington University professor of chemistry in Arts & Sciences, is doing this so that the electrodes on the chip can be used to monitor the biological behavior of up to 12,000 molecules at the same time.
Lock and key
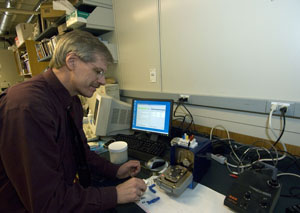
The work is motivated by a desire to map the three-dimensional requirements of biological receptors on cell surfaces. Typically, receptors bind small molecules through a lock and key mechanism where the molecule is the key and the receptor the lock. The nature and shape of molecules that serve as keys tells about the binding requirements of the receptor. Traditionally, probing a receptor this way has been done by making a library of molecules, treating it with the receptor, washing away any excess receptor that has not found a key, and then treating the bound receptors with an antibody that recognizes the receptor and is tagged to a fluorescent label. The washing step risks removing a bound receptor if it does not bind the molecular key strongly enough. But, with an electrochemically addressable computer chip, provided in great abundance by one of his sponsor’s, CombiMatrix in Seattle, Moeller saw a way of probing the binding of a library with a receptor without the need for washing by putting each member of the molecular library by an electrode that can then be used to monitor its behavior.
The electrochemically addressable chips being used represent a new environment for synthetic organic chemistry, changing the way chemists and biomedical researchers make molecules, build molecular libraries and understand the mechanisms by which molecules bind to receptor sites.
“We believe we can move most of modern synthetic organic chemistry to this electrochemically addressable chip. In this way, a wide variety of molecules can be generated and then probed for their biological behavior in real-time,” said Moeller. “It’s a tool, still being developed, to map receptors. We’re right at the cusp of things.”
Moeller published on the technology in a recent article in the Journal of the American Chemical Society, Vol. 28 16020, 2006. He will discuss the work at the 211th National Meeting of the Electrochemistry Society in Chicago, May 7, 2007. The National Science Foundation and the CombiMatrix Corp. in Seattle fund the work.
The Great Wall of China syndrome
Moeller said that the standard problem for synthetic organic chemists has typically been a structural one — how do we build molecules having novel structures?
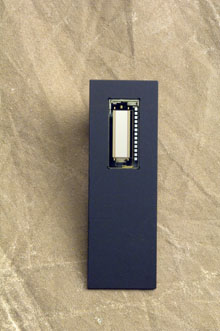
“We’ve worked very hard to develop new chemical reactions that allow us to make new structures that are either difficult or impossible to make with the synthetic tools available,” he said. “But now for the first time it’s not just a matter of structure, but rather a matter of location and scale — a logistical problem.”
He offered the Great Wall of China as an example of a classic logistics problem — the structure itself was simple, but getting the tools and manpower necessary for the task to the remote regions it was built in on the scale necessary was “a logistical nightmare.”
Getting 12,000 electrodes per square centimeter to selectively do your chemical bidding is Moeller’s logistical nightmare. How do you get chemical reactions to happen at just one of the electrodes? Here’s how he and his colleagues addressed it.
Scientists at CombiMatrix initially pioneered an approach for covering the chip with a polymer, attaching a substrate to the polymer right above the electrode, and then using the electrode to initiate a chemical reaction that modified the substrate and converted it into a product. Putting a confining agent into the solution destroys the reagent made, keeping it from going to another electrode. This was done for the generation of acids and bases and applied to the synthesis of DNA and peptide polymers. But could this approach be used to make the more difficult to synthesize shaped molecules needed for mapping a receptor? This would require a diverse array of reactions to be compatible with the chips, particularly reactions that capitalized on modern, state-of-the-art metal-based reagents.
Working with his students, Moeller started by running a well-known electrochemical Wacker oxidation reaction, which converts an olefin into a ketone using palladium(II) on selected electrodes on the chip. In order to do this, the palladium(II) reagent needed at a selected electrode was actually made at the desired site using the electrode. This allowed for the desired reaction at only electrodes that were turned on. To keep the palladium(II) from going to a neighboring electrode a second reagent was added to the solution above the chip that destroyed the palladium(II) reagent. In this way, nothing happened at electrodes that were not being used.
Reversing the polarity of things
“The chip is an ongoing battle between active reagent and inactive reagent,” Moeller said. “The active reagent is fine — it does the chemistry I want it to do — but as soon as it gets away from the turned-on electrode the reaction that inactivates it needs to take over.”
With an overall strategy in place, Moeller and his collaborators are working to expand the scope of reactions that can be done. For example, they have carried out the oxidation of an alcohol to form a carbonyl and then used the carbonyl to put a dye down. They placed a green dye at alternating electrodes in a checkerboard pattern, and then used the alternate set of electrodes to put down a red dye. The result was a red and green chip, Washington University’s colors. This, Moeller said, is a striking image of the technique’s ability to do chemistry at specific electrodes.
“What electrochemistry does best is to reverse the polarity of things,” Moeller said. “It takes things that are electron-poor and makes them electron-rich and vice versa; it can take an oxidant and turn it into a reductant; a reductant into an oxidant; a base into an acid and an acid into a base; and so on. In the end, almost any chemical reagent can be made at an electrode and used on the chips.”
Moeller said that , along with emphasizing new reactions, he and his collaborators are working to better identify the molecules they make on the chips, and perfect the signaling techniques used to monitor molecules on the chips. He praised the time-of-flight secondary-ion-mass spectrometry work of his Washington University colleague Amy Walker, Ph.D., assistant professor of chemistry, in the monitoring of his system.