There’s no organ system in the body that does as much for humans as roots do for plants. Part anchor and part mouth, a plant’s root system architecture is critical to its success. But the process of growing new roots is costly to a plant, and there can be diminishing returns.
It’s not clear-cut how a plant determines enough is enough and stops making roots. New research from Washington University in St. Louis identifies a cellular transporter that links two of the most powerful hormones in plant development — auxin and cytokinin — and shows how they are involved in putting the brakes on root initiation and progression. The new work by Lucia Strader, associate professor of biology in Arts & Sciences, and her co-authors is published July 18 in the journal Developmental Cell.
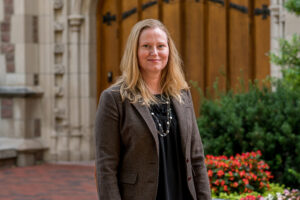
“This is exciting because for a long time, we’ve known that auxin and cytokinin have opposing roles, but the direct links between how one of them might affect the other in lateral root production was not well understood,” Strader said.
Lateral roots are the roots that branch out horizontally like fingers stretching out to the side. They make up the majority of root mass.
“Our data suggests that one of the ways that cytokinin can decrease lateral root production is by increasing the levels of this transporter to limit contributions of this particular auxin precursor to active auxin,” she said.
Pumping and releasing the brakes
The plant hormone auxin controls almost every aspect of plant growth and development, including stimulating root growth overall. Previous research has shown that another important hormone called cytokinin has a limiting effect — controlling the locations where new lateral roots could possibly sprout, and ensuring sufficient spacing between neighboring roots.
Until now, however, scientists have not identified how these hormones “talk” to each other.
Working with the model plant Arabidopsis thaliana, Strader decoded a key to this conversation.
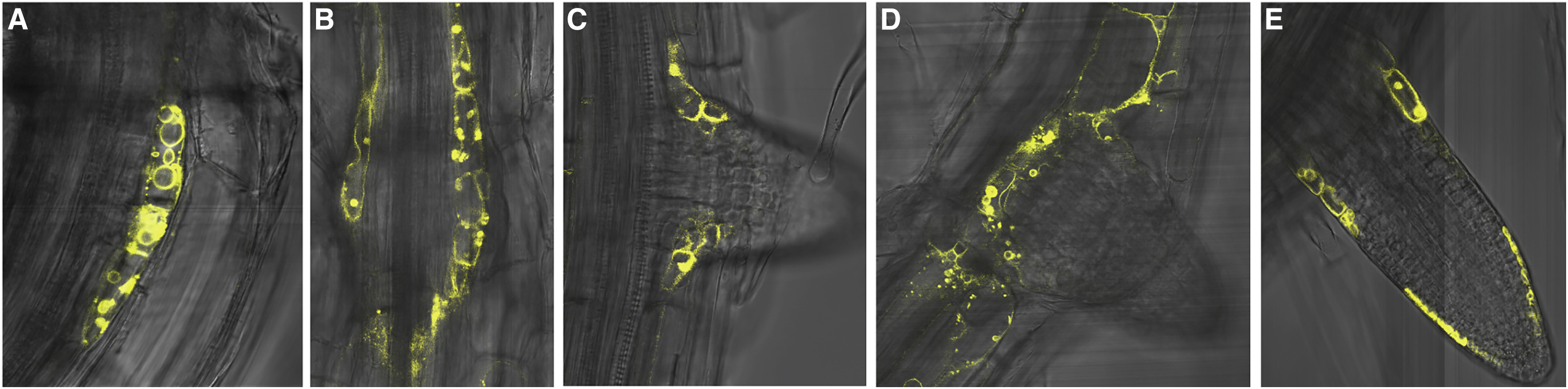
Strader discovered that a cellular transporter she dubbed TOB1 can stash away an auxin precursor by moving it into a vacuole, an organ in the plant cell that acts as a kind of storage space or holding pen. That action prevents the precursor, which is called IBA, from metabolizing into full-fledged auxin — with all of its root-promoting capabilities.
“If TOB1 is the brake, cytokinin is the foot on the brake,” Strader said. “It’s the thing that’s saying how much TOB1 should be around to dampen down on lateral root production.”
Plants can increase or relax the hold as needed. Strader and her team used gene modification techniques to eliminate the transporter, and saw dramatic effects in the next generation of plants.
“When you get rid of this transporter, you have about double the number of lateral roots as the wild type, without sacrificing root depth,” Strader said.
Strader also repeated certain of her experiments with a yeast and frog oocytes (with the help of Wolf B. Frommer of Heinrich Heine University in Düsseldorf, Germany) instead of a plant, and found TOB1 to be just as effective as a transporter for IBA in these systems.
Slow but steady may be better for plants
Strader’s research shows how she and her collaborators started with an unbiased genetic screen and ended up identifying a critical regulator of an aspect of plant development that often gets ignored — one that’s not readily visible, because roots are underground.
And Arabidopsis roots are tiny. At two weeks old, its leaves are much smaller than a dime, and its roots are thread-like and transparent. To bring the process of lateral root production into the daylight, Strader reached out for help from Christopher Topp, a principal investigator at the Danforth Plant Sciences Center. Topp used a non-destructive technique to capture the first ever three-dimensional serial images of developing Arabidopsis plants in medium — a feat that is remarkable, given how much research has been conducted with this model plant.
Selected 3D reconstructions of 25-day-old WT (Col-0) and tob1-1 plant, with each datapoint color coded. The tob1 displays more emerged lateral roots than wild type at every examined time point. (Video by Christopher Topp, Courtesy Developmental Cell)
What they uncovered is useful because the molecular mechanisms regulating root architecture are critically understudied.
Understanding why and how plants make different types of root architectures can help to develop plants that better cope with distinct soil conditions and environments. In follow-on work, Strader has already begun to look at how TOB1 mutants respond differently in soils with different micronutrients.
“When you get rid of your brakes, you just go crazy,” Strader said. “In the beginning, this looks like a good agricultural trait. You want all of your plants in the soil to explore more soil to get more nutrients, to get more roots to the water.
“But, if you don’t ever have a brake, you waste your time making more and more of these,” she said. “Probably at some stage in the plant’s life cycle, this slow but steady approach is better than the all-out, ‘let’s make lateral roots everywhere,’ one.”