An accurate analog clock tick-tick-ticks with a constant precision and well known frequency: one tick per second. The longer you let it tick, the better to test its accuracy — 10 times as long corresponds to a ten-fold improvement in any frequency uncertainty. But is there a faster way to determine a frequency?
It turns out there is, in a new discovery published this week in Physical Review Letters through a collaboration between Washington University in St. Louis and the University of Rochester.
The speed-up in frequency measurement comes from quantum mechanics. When a quantum bit is used to measure the frequency of a signal, the strange rules of quantum mechanics allow the frequency measurement to be much more accurate. The technique hinges on the ability to put the quantum bit in a superposition of its two quantum states, and then shift these states around in time with the signal.
Kater Murch, assistant professor of physics in Arts & Sciences at Washington University, along with Arts & Sciences graduate student Mahdi Naghiloo and theory collaborator Andrew Jordan of the University of Rochester described the technique as a “quantum magic trick.”
“It’s reminiscent of the magic tricks that involve a ball placed under one of two cups and the cups are shuffled around — except this time, the ball can be under both cups at the same time,” Murch said. “The resulting speedup in frequency measurement is astonishing. Now, by measuring for 10 times as long, the frequency uncertainty can be reduced by a factor of 100 — enabling enhanced resolution of the frequency beyond any other technique of its kind.
“Earlier theory work published by the Jordan group this year has proven in two separate papers that the technique applied in this paper is the theoretical optimum that quantum mechanics allows.”
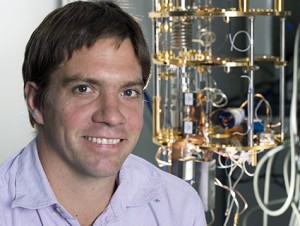
The experiment was completed by using a superconducting quantum system where an external oscillating signal with unknown frequency caused the quantum system to undergo periodic changes. By applying quantum pulses on top of the oscillating signal, the state of the system could be controlled so that the final readout of the quantum system became highly sensitive to the precise value of the oscillation frequency. The underlying physical source of the advantage is related to the fact that the energy of the quantum system is time-dependent, which causes the quantum states corresponding to different frequencies to accelerate away from each other, giving enhanced distinguishability in a given time.
This method permitted enhanced resolution of the frequency beyond any other technique of its kind, Jordan said.
This work is just one example of how the new field of quantum technologies uses the laws of quantum physics for technological advantage over classical physics, Jordan said. Other examples include quantum computing, quantum sensing and quantum simulation. For those fields, the exploitation of quantum physics provides benefits such as a speed up of database search, the factoring of large numbers or the rapid simulation of complex molecules.
Such fine-scale measurement of the frequency of a periodic signal is the fundamental ingredient in diverse applications, including MRI medical imaging devices, the analysis of light emitted from stars and, of course, clock precision. Accelerating these measurements in a way that Murch and Jordan have demonstrated could have profound impacts in many areas.
Murch and Naghiloo used timekeeping and GPS, and such constantly advancing technologies, as examples of the importance of their findings.
“Nowadays, most of us carry a phone in our pocket that is capable of telling us almost exactly where we are on Earth using the Global Positioning System,” Murch said. “The way this works is that your phone receives signals from several different satellites, and by timing the relative arrival of these signals it infers your position. The accuracy of the timing directly relates to the accuracy of your position — a relationship between timekeeping and navigation that has persisted for hundreds of years.
“Well before GPS, a sailor who wanted to know his location would navigate by the stars. In the Northern Hemisphere, the height of the north star will tell you your latitude, but to know your longitude, you need to keep track of the time. As the night goes on, the stars circle around the north star — the height of any star above the horizon is related to the local time, and by comparing this time to a clock set to Greenwich Mean Time, the time difference gives your longitude.”
Nautical timekeeping underscores the vitality of frequency advances.
“In the 1700s, accurate clocks were the main limitation to ocean navigation,” Murch said. “The Scilly naval disaster of 1707 — one of the worst disasters in British naval history — was widely blamed on poor navigation, prompting the British government to invest heavily in precise clocks. The resulting chronometers transformed marine navigation and greatly accelerated the age of discovery.
“Advances in timekeeping continue to have profound impact on technology and fundamental science. Quantum tools, such as the quantum speedup in frequency measurement that we discovered, are necessary to push these technologies forward. This is an exciting time for quantum physics because these quantum resources are increasingly leading to practical advantages over traditional measurement approaches.”