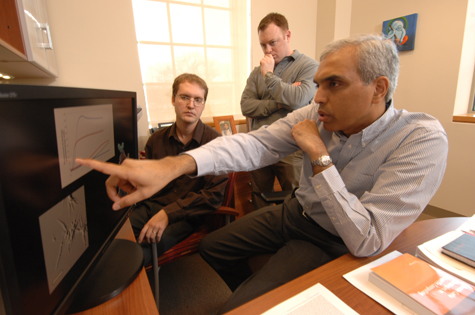
If you open any biology
textbook to the section on proteins, you will learn that a protein is
made up of a sequence of amino acids, that the sequence determines how
the chain of amino acids folds into a compact structure, and that the
folded protein’s structure determines its function. In other words
sequence encodes structure and function derives from structure.
But
the textbooks may have to be rewritten. As Rohit Pappu and two
colleagues explain in a perspective published Sept. 20 in Science, a
large class of proteins doesn’t adhere to the structure-function paradigm.
Called intrinsically disordered proteins, these proteins fail fold either in
whole or in part and yet they are functional.
We sat down recently with
Pappu, PhD, professor of biomedical engineering and director of the Center
for Biological Systems Engineering at Washington University in St. Louis
to catch up on the latest science.
When did people realize some proteins violate the rules?
It’s been about 20 years. The earliest clue was that some protein segments didn’t show up in X-ray crystallography or NMR studies, the standard ways of studying protein structure.
By the 1990s people who studied how proteins interact with DNA had noticed the proteins often change shape when they interact with DNA. In the absence of DNA all the standard probes for protein structure reported back that the proteins were floppy, and yet when the protein formed a complex with DNA it had a well-defined three-dimensional structure.
How did you first come to hear about them?
By serendipity. When I was leaving Johns Hopkins University to come to Washington University in 2001 I had a meeting with Keith Dunker of the Indiana University Schools of Medicine and Informatics, one of the founding fathers of this field. It was pure chance.
The meeting started awkwardly because Keith was wondering who I was and I had never heard of him. I was working on a polymer physics description of unfolded proteins, and it turned out he had just written an 80-page review paper on intrinsically disordered proteins.
“Every time you talk to people in the back alleys of protein science,” he said, “they tell you their proteins are very flexible or highly dynamic, and this dynamism is important for function.”
So Keith did two things. He synthesized all of the information then known about these flexible, highly disordered proteins. And, together with his colleague Vladimir Uversky, he asked if it was possible to predict which sequences would be incapable of folding autonomously.
With the help of computer scientists who taught him how to look for patterns in high-dimensional spaces, he learned that 11 out of the 20 amino acids predispose sequences toward being disordered. Today there are about 20 predictors of disorder.
So when I heard this story I thought, “OK, either this is absolutely crackers or it is going to be transformative. I’m going to take a bet on transformative because I find what he’s saying compelling.”
So during my first two years at Washington University I started to devour the literature. I think I scared a lot of people here who weren’t sure they had hired the person they thought they were hiring.
What percentage of proteins are intrinsically disordered?
It goes by kingdoms. So in bacteria and prokaryotic organisms these numbers are pretty small. They’re about 5 percent of the proteome, the entire set of proteins made by an organism. But if you go to eukaryotes or multicellular organisms then the numbers get to 30 or 40 percent of the entire proteome.
But if you ask what percentage of sequences that make up the signaling proteome — proteins that are busy passing messages to other proteins — are intrinsically disordered, then the numbers jump up to 60 to 70 percent.
There seems to be a division of responsibilities. Structured proteins take part in catalysis and transport. Intrinsically disordered proteins are important for signaling and regulation.
Why are disordered proteins involved in signaling and regulation?
I think there are two logical reasons. One is that complexes involving intrinsically disordered proteins are short-lived and the other is that they typically bind many rather than just one molecule.
If a molecule cannot fold except in the context of a complex, then some of the energy used for folding must come from intermolecular interactions. And if the molecule has taken out an energy loan, the complex that forms is not going to be very stable or long-lived.
You’re combining high specificity (because the protein will only fold when it recognizes the molecule with which it forms a complex) with low overall affinity (because the complex is not very stable).
The many-to-one interactions arise because disordered proteins typically function through short amino acid stretches instead of large protein-protein interfaces. So a single polypeptide stretch can interact with multiple targets. One motif talks to one protein, and a second motif talks to another protein, but through the chain they can communicate with each other.
That’s why these molecules happen to be at hubs within networks. They’re trafficking information through networks like the air traffic control tower in an airport hub.
Because most of their functions are carried out by these very short motifs, they are capable of coordinating large amounts of information that are disparate in nature. You get many things happening at the same time.
What was remarkable to me about your perspective is that you emphasized functionality of these proteins. Isn’t the name a bit misleading?
You’re right. As we get to know them better we’ve thought we should have called disordered proteins, molecular rheostats.
But to a physicist disorder just means thermal fluctuations are dominant, so for physicists it’s an accurate description. The problem is that in the biomedical field the word disorder has been coopted for disease.
You mention several tricks these proteins have up their sleeves. One I thought was clever was modulating the local chemical environment to encourage a particular reaction.
This is a very important idea. If you’re doing chemistry in a test tube, and you want to make a reaction go, you increase the concentration of the reactants: A needs to bump into B and do so often. But this is a matter of probability so you might need a gazillion molecules of A and bazillion of B to get some statistics.
But if there’s a tether between A and B, they’re guaranteed to bump into each other quite often. You might be able to get away with a handful of molecules instead of gazillion.
The loose tether, in effect, increases the concentration of A around B, and the tether is often a disordered region.
Another thing you mentioned was cryptic disorder: the idea that structured proteins can become disordered. That’s such a backward flip.
Richard Kriwacki of St. Jude Children’s Research Hospital, a co-author of this perspective, is the person who made the clearest discovery in that regard. He shows that two structured domains can come together — this is part of the whole p53 tumor suppressor apparatus — and in trying to commingle, they undergo an unfolding transition that exposes sites that otherwise were buried.
This is the idea of cryptic disorder: that domains, by promoting disorder in one another, reveal hidden, or cryptic, motifs or sites that are now available for function.
You mention that in the biomedical community disorder is associated with disease. Your co-author M. Madan Babu of the MRC Laboratory of Molecular Biology in Cambridge has written about this connection.
Yes. Cells make many decisions. They decide to differentiate, to die, to regenerate, or to go quiet ,and these decisions are controlled by regulatory networks. The integrators in the networks are predominantly disordered regions.
So the question is: Will mutations in those regions give rise to unwarranted cellular phenotypes and hence diseases, such as cardiovascular disorders, cancer and neurodegeneration? The answer is absolutely yes.
But what we are learning is that mutations in disordered regions don’t necessarily generate a deleterious phenotype because disordered regions are fairly unconstrained compared to structured regions. So these regions are also engines of robustness.
They’re more robust to mutation?
At least the one study that looked at cancer mutation would suggest that. It showed that cancer-associated mutations partition toward structured regions of proteins, not toward disordered regions.
Do you think the new awareness of disordered proteins will lead to medical breakthroughs?
Maybe. If I tell you that a disordered protein is at the hub of a network, then it stands to reason that targeting the hub with a drug gives you a ready-made way of controlling a cellular decision.
The only problem is that we don’t quite know what it means to target a hub. If a protein has a very precise shape we know how to target it: it’s like designing a key for a lock. But if a protein is disordered we have to understand what that means for that particular hub. We also have to be aware that anything that changes the hub will change a range of downstream processes, pathways and cellular decisions.
Nonetheless many people now are talking about these disordered proteins as druggable targets.
Together with Peter Tompa, another of the field’s founders, you organized a Gordon Research Conference on disordered proteins this summer. This was a chance for the leading scientists in the field to explore their thoughts off the record. What was the consensus?
It was evident that the more you know the harder it is to draw the order/disorder demarcation. There is a continuum. In fact, many disordered regions do end up adopting structure; they just defer the adoption of structure to the appropriate context.
We wrote the Science perspective because we thought this was the opportune moment to make the point that there is probably evolutionary synergy between the structured domains and the disordered regions, and that synergy is what we really need to wrap our heads around if we are really going to get at how biology integrates signals to control processes and generate responses.
So at the end of the day what you end up with are molecular integrators and it appears that these disordered regions are the molecular integrators.