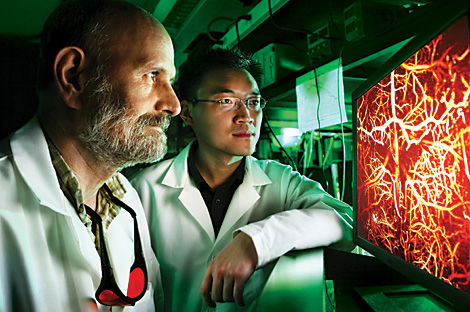
For years, the field of optical imaging in biological tissue had languished, with few advances and no significant growth. The concept was promising — using light to image organs, cells, and blood vessels, noninvasively and without any radiation — but it seemed impossible to obtain high-resolution images at any significant depth. Then came Lihong V. Wang’s seminal paper, which appeared in the July 2003 issue of the journal Nature Biotechnology.
“2003 is the magic number,” says Wang, Ph.D., now the Gene K. Beare Distinguished Professor in the Department of Biomedical Engineering. “We published the first paper on functional imaging using photoacoustics. That excited everybody and attracted newcomers to the field.”
Today, Wang has a chart showing the growth in research worldwide since the publication of his paper, the most cited in his field. In 2003, only some 30 presentations were made on photoacoustic tomography in the largest conference on this topic, but through the following years, that number rose dramatically: to 40 by 2004, 80 by 2007, and 132 in 2010. Wang and his lab were the founders of a new area of scientific inquiry — one that combined light and sound to create a new form of functional imaging — with many potential applications for cancer research.
“Lihong’s pioneering work is a wonderful example of an innovative combination of physics,engineering, and medicine,” says Frank C.P. Yin, M.D., Ph.D., the Stephen F. and Camilla T. Brauer Distinguished Professor of Biomedical Engineering. “Its high temporal and spatial resolution will enable us to obtain structural and functional information more safely, cheaply, and easily than with any existing method. I predict that it will revolutionize our approach to diagnosing and treating diseases and produce as-yet-unimaginable benefits to humankind.”
In an unfavorable economic climate, Wang’s work has attracted significant funding, particularly from the National Institutes of Health (NIH). Over the past three years, he and his group have received some $15 million in grants, with another $1.9 million in the offing.
Often, they collaborate with physicians — breast surgeons, radiologists, GI specialists, and dermatologists, among others — from the Washington University School of Medicine.
“The collaboration between Mallinckrodt Institute of Radiology (MIR) and the Department of Biomedical Engineering has been terrific,” says Gilbert R. Jost, M.D., Elizabeth Mallinckrodt Professor of Radiology and Director of MIR. “By working at the medical school, Lihong Wang has been able to explore important clinical applications of his new imaging technique.”
Wang himself is invited to give frequent talks on photoacoustic tomography across the United States and around the world: in Japan, Turkey, China, Belgium, and Canada last year alone. “Every time I give a talk, I find new potential collaborators or users,” says Wang, who also has joint appointments in MIR and in Electrical & Systems Engineering. “They are interested in these technologies to solve their own research problems.”
Using Light for Imaging
While scientists have long been interested in using light for imaging, the nature of light made that difficult. Fire a laser pulse into tissue, and the light particles continue on a straight path for only a short distance, usually less than 100 microns — approximately the width of a hair.
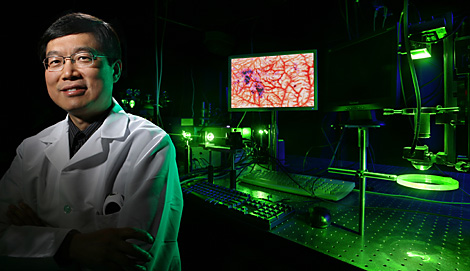
“Then they encounter something, such as cell nuclei, and get scattered,” says Wang. “Photons just bounce around, making it challenging to achieve high-resolution imaging at depths beyond approximately 1 mm.”
After they scatter, however, the photons continue to penetrate tissue, up to a depth of around 5 centimeters (about 2 inches). That would make it possible to image a compressed breast, for example, and check for tumors. But how to harness the errant photons and retrieve the critical spatial information?
“If you want to image a piece of relatively thick tissue and see the middle plane, which is the hardest plane to image, the illumination and detection photon paths will both be tortuous,” says Wang. “That means any feature in the middle plane will be blurred out.”
Photoacoustics is Born
When the field of photoacoustics first developed, it had no relation to imaging. In June 1880, Alexander Graham Bell tested a “photophone,” an alternative to the telephone. Bell’s idea was to encode sound into a light beam, propagate the beam in air, then convert the beam back to sound again. However, this phone required “line of sight” transmission, and the idea proved impractical.
The idea of adapting photoacoustics to imaging came along during the 1980s, when some industry scientists began using this technique for non-biological-materials testing. At that point, they were hunting for sub-surface cracks in metal or ceramic materials. How to extend this concept to three-dimensional structures?
In Wang’s 2003 paper, he and his colleagues showed that photoacoustic imaging could provide detailed images of internal structures and overcome the problem of photon diffusion. With extraordinary clarity, their images of an animal brain depicted blood vessels and brain function. They had higher resolution than any other optical images of the brain ever obtained before.
“Imaging scientists were convinced that photoacoustics really makes a difference,” says Wang. “This was the right way to go.”
What was Wang’s approach? Converting light into sound, which scatters 1,000 times less than light — and thus produces a dramatically improved optical image.
First, the laser sends light pulses into tissue; as it is absorbed, it generates a minute temperature rise, which creates an ultrasonic sound wave detectable by an ultrasound transducer. The location and size of the target structure are calculated by computer, using a sophisticated triangulation technique.
“You are essentially listening to an optical structure,” Wang says. “You’re hearing the picture instead of looking at it.”
With this method, they can provide a look at many different aspects of physiology: hemoglobin, melanin, fat, even water in tissue. Thus, unlike X-ray imaging, photoacoustic imaging is not just structural imaging; rather, it is also functional imaging, able to tell whether tissue is alive and functioning properly by measuring its physiological parameters.
With this information, physicians will be better able to detect cancer early, says Wang. For instance, photoacoustic imaging can measure the oxygen saturation of hemoglobin, which correlates with hypermetabolism, a hallmark of cancer. The same thing is true of the concentration of hemoglobin, which correlates with angiogenesis — another predictor of cancer.
Photoacoustic tomography can also do molecular imaging that detects biomarkers such as integrins, a family of receptor proteins that regulate cell growth and tend to over express in cancer. Further, it can detect gene expression: Is a particular gene being expressed in cancer? By pinpointing which gene it is, can we target therapy more precisely?
Overall, this imaging method has a number of advantages over existing modalities. It is nonionizing radiation, so it does not carry the risks of radiation. It provides clear, high quality images. To achieve deeper penetration, scientists can substitute radio-frequency waves for the light waves. Further, this is a “scalable method,” says Wang.
“Using photoacoustic tomography, we can image shallow, but we can also image deep: from subcellular organelles through individual cells all the way to organs. It is the only functional imaging modality that allows us to image over so many levels of tissue structure, and that can potentially accelerate the translation of microscopic laboratory discoveries to macroscopic clinical practices.”
Wang’s Background
Wang, a native of Guangshui in Hubei province, China, received bachelor’s and master’s degrees from Huazhong University of Science and Technology. Then he moved to the United States and earned a doctorate in electrical engineering from Rice University in 1992.
He worked as a postdoctoral fellow and later as an assistant professor at the University of Texas M.D. Anderson Cancer Center. Next, he joined the faculty at Texas A&M University, and by the time he left in 2006, he was the Royce E. Wisenbaker II Endowed Professor of Biomedical Engineering and Electrical Engineering.
As a postdoctoral fellow, he became interested in imaging — but how could he get a good image using light alone? So he began thinking about different ways of combining ultrasound and light, and his research team created a model of photon transport in scattering media, called the Monte Carlo method, which is widely used today.
Coming to Washington University meant that he was much closer to a medical center than he had been at Texas A&M, where the nearest teaching hospital was 90 miles away. “Washington University is able to provide an excellent environment for our translational research,” he says.
Today, he has a 30-person group — the largest photoacoustic tomography laboratory in the world — with more than 50 percent postdoctoral fellows or faculty researchers and the rest Ph.D. students. The lab built its own photoacoustic equipment, including multiple three-dimensional microscopes; some of this technology has been licensed to outside companies for commercialization.
He works with fellow biomedical engineering faculty members, including Younan Xia, Ph.D., James M. McKelvey Professor, also in the Department of Biomedical Engineering, whose laboratory invented light-absorbent gold nanocages. Together, Wang and Xia have applied these nanocages and photoacoustics to map sentinel lymph nodes noninvasively, thus reducing a patient’s exposure to radioactivity.
Through the years, he has garnered awards for his work, including the NIH FIRST Award and the National Science Foundation CAREER Award. He chairs the International Biomedical Optics Society, and is newly appointed editor-in-chief of the Journal of Biomedical Optics. He has written two textbooks, including one of the first in his field, Biomedical Optics: Principles and Imaging, which recently received the Goodman Book Writing Award from SPIE and the OSA — and some 206 peer-reviewed journal articles.
Looking five years into the future, Wang says he would like to see some giant strides forward in clinical applications with the help of photoacoustic imaging: melanoma screening and imaging, GI and colon cancer detection, neonatal brain imaging, breast cancer screening and imaging, transrectal prostate cancer detection, and possibly even low-cost brain cancer screening.
“Another application that is high on my list is early prediction of response to chemotherapy,” says Wang. “Sometimes a cancer patient goes through the entire eight-week cycle of chemotherapy only to find that this was not the right drug.
If we could detect the efficacy within the first two weeks, we could save a lot of time and potential side effects for the patient.”
Of course, there are still hurdles to overcome. Right now, most of these applications are currently being tested in animals, with human trials to follow.
And they need to solve the problem of imaging through thick bone, such as the adult skull, which attenuates the ultrasound signal and distorts the image.
But the outcome of their work, they hope, will be a healthier world.
“Prevention of cancer would be the best solution,” says Wang, “but the second-best solution is early detection, because if cancer is identified early, it can likely be cured. Currently, we cannot prevent cancer, so we need to focus on early detection.”
This article appeared in the Spring 2010 issue of Momentum magazine.