In recent years, genetic studies have uncovered hundreds of DNA variations linked to common diseases, such as cancer or diabetes, raising the prospect that scientists can gauge disease risk based on information in an individual’s genome. But the variations identified to date only account for a small percentage – typically one to three percent – of the overall genetic risk of any common disease.
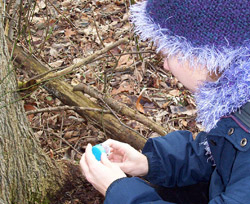
This disappointment has led geneticist Barak Cohen, Ph.D., of Washington University School of Medicine in St. Louis, to suggest that scientists need to get a better handle on the ways genes interact to influence disease risk.
“For diseases that are major health problems, many different genetic variants combine to affect an individual’s risk,” says Cohen. “The problem is that we as scientists are really lousy at predicting how these variations interact to determine whether an individual is likely to develop a common disease or respond to a particular drug.”
This reality begs the question: Is it possible to tease apart a complex genetic trait to reveal the precise genetic variations that have combined to produce it? Yes, Cohen and his group report in the Jan. 23 issue of Science. If the research can be replicated, it suggests that scientists need better statistical models and other tools to understand genetic interactions.
The researchers turned to a simple organism, the yeast Saccharomyces cerevisiae, culled from North American oak trees and vineyards, where it grows naturally, to find their answer.
“This was a test case,” Cohen explains. “If we can’t dissect a complex genetic trait that occurs naturally in yeast and show how multiple genes interact to produce a particular trait, then there’s no hope for doing it in humans.”
The researchers probed the genome of yeast to find the DNA variations that determine the efficiency with which the yeast undergo sexual reproduction, a process called sporulation. Cohen acknowledges it’s not a particularly fascinating trait, but it is one that can be measured easily and precisely.
“We don’t have any particular fondness for sporulation,” he says. “We are simply using it as a model to understand how multiple genes interact to influence variation in a biological process. Our hope is that a complex trait is put together in yeast in a similar way as it is in humans.”
When it comes to sporulation, the yeast from the oak tree samples produce spores with 99 percent efficiency; the vineyard strains are far less efficient, at seven percent.
The scientists discovered that just four variants, or single nucleotide polymorphisms (SNPs), in three yeast genes account for nearly 90 percent of the genetic contribution to sporulation efficiency. By moving each of the four variants from one yeast strain to the other, they produced oak strains that sporulated like vineyard strains and vice versa.
“To put this into context, there are about 85,000 SNP differences between the two strains of yeast, and by moving just four of them, we effectively reversed the phenotype of the two strains,” Cohen says.
The researchers also exchanged every combination of the four SNPs between the yeast strains to determine how the genetic variations interacted. Interestingly, any two or more of variants from the oak tree strain increased sporulation efficiency far more than would have been expected based on the individual contributions of each SNP.
“The variations interacted like crazy,” Cohen says. “The combined effects of variants were always larger than the sum of their individual effects.”
Understanding these interactions was critical for the scientists to accurately predict how a strain would behave based on the variations it carries in its genome. “Only by accounting for the interactions between variants could they predict how particular variants combine to increase or decrease sporulation.”
The researchers also were surprised to discover that the four SNPs occurred in genes known as transcription factors, which have the ability to turn on other genes. This finding lends weight to the emerging theory that transcription factors may be a rich source of meaningful genetic variations, he says.
“It’s a big genome with many different types of genes,” Cohen says. “The probability that all four SNPs would be in transcription factor genes is very, very low. This suggests transcription factors may be more likely to harbor significant variations than other classes of genes.”
Cohen acknowledges that dissecting a complex genetic trait in humans is far more difficult due to the sheer number of SNPs in the human genome. But his research suggests that scientists need a better understanding of genetic interactions so that information in the human genome can one day accurately predict the diseases an individual is susceptible to and a list of drugs that are most effective for that individual. In other words, a new era of personalized medicine.
Washington University School of Medicine’s 2,100 employed and volunteer faculty physicians also are the medical staff of Barnes-Jewish and St. Louis Children’s hospitals. The School of Medicine is one of the leading medical research, teaching and patient care institutions in the nation, currently ranked third in the nation by U.S. News & World Report. Through its affiliations with Barnes-Jewish and St. Louis Children’s hospitals, the School of Medicine is linked to BJC HealthCare.