Researchers at Washington University in St. Louis have shed light on a basic process that could improve future solar cells.
“One type of solar cell design starts with a chain of chromophores strung between two electrodes,” explained Dewey Holten, Ph.D., professor of chemistry in Arts & Sciences. “This chain absorbs the light energy and directs that energy toward one electrode, where it is deposited as an electron. The molecule that lost the electron now has a positive charge left behind, called a hole. The hole migrates down the chain toward the opposite electrode. The electron and the hole recombine in the external circuit, creating an electrical current to do work.”
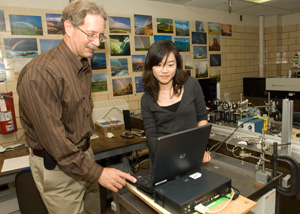
Holten and graduate student Hee-eun Song have directly measured the rate of hole transfer between identical porphyrin compounds in their ground states. These results are key to understanding the fundamental processes underlying charge separation in this sort of structure and have applications for improving the efficiency of solar cells.
Their results represent the first time that ground-state hole transfer rates have been precisely measured. Previously, hole transfer in chains of porphrin molecules was known to take from 20 picoseconds to 50 nanoseconds, a range that spans three orders of magnitude. These studies have defined the time as 0.5-1 nanosecond.
The work has been published in the Journal of the American Chemical Society and is in press at the Journal of Physical Chemistry B and Photochemistry and Photobiology. The Department of Energy Solar Photochemistry Program provided funding.
Hopping downhill
Thermodynamic stability is often the driving force for the hole to move down the chain. However, this requires that the chain be made up of a variety of molecules so that each hop is downhill in energy.
“From a synthetic standpoint, it is easier to build a string of similar molecules with a minimum number of energy gradient steps built in. The challenge then becomes monitoring hole transfer between identical molecules,” said Holten.
The multiporphyrinic arrays they studied were synthesized by a group at North Carolina State University under the direction of Jonathan Lindsey, Ph.D., Glaxo Distinguished University Professor of Chemistry.
Holten and his group placed the molecular arrays in a predefined starting form by electrochemically oxidizing one of the porphyrins to generate the hole and exciting another with light to make an electronic excited state. Then they used ultrafast femtosecond timescale transient absorption spectroscopy to monitor the hole transfer process between equivalent porphyrins.
“We compared the spectroscopic and kinetic results for the monomer, dyads, and triads,” Holten said. “From this information, we could back out the rates of hole transfer between the equivalent sites.”
David Bocian, Ph.D., professor of chemistry at the University of California, Riverside and his group performed additional spectroscopic studies.
“This work is an example of how an exciting collaboration between scientists can produce results that are fundamentally important, develop a general experimental method that can be adopted by other scientists, and also have real world applications,” said Holten.