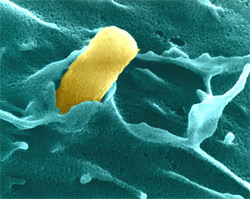
New insights into the bacteria responsible for urinary tract infections appear to open up an opportunity for disabling a wide range of bacteria.
In a study published in the Proceedings of the National Academy of Sciences, researchers at Washington University School of Medicine in St. Louis reveal how a protein known as PapD helps E. coli assemble a pilus — one of several sticky fibers, or pili, that allow the bacterium to latch onto and infect host cells.
Scientists are using what they’ve learned to begin designing pilicides, new treatments that stop pili construction and disrupt the infection process.
Many pathogens use similar processes to form pili, notes senior investigator Scott Hultgren, Ph.D., the Helen L. Stoever Professor of Molecular Microbiology.
“In proteins like PapD that are used for these pilus formation processes, the region of the protein responsible for its activity changes very little if at all,” Hultgren explains. “That gives us hope that any pilicide we develop may be useful against a broad spectrum of bacteria.”
Hultgren’s lab studies the complex arsenal of tools and tricks employed by E. coli during urinary tract infections (UTIs). E. coli is thought to be responsible for 80 to 90 percent of all UTIs, which mainly occur in women and are the second most common bacterial infection. Scientists estimate half of all women will experience a UTI at some point in their lives, and recurrent UTIs will affect 20 to 40 percent of those patients.

Hultgren’s lab had shown that PapD was essential to E. coli’s ability to assemble several proteins together to make one type of pilus. To get exact details of how PapD contributed to this assembly process, Hultgren’s group collaborated with the lab of protein folding expert Carl Frieden, Ph.D., Raymond H. Witcoff Professor and head of Biochemistry and Molecular Biophysics at Washington University School of Medicine. Postdoctoral fellow James Bann, Ph.D., was lead investigator for the project. Bann is now assistant professor of chemistry at Wichita State University.
Changes in the way a protein is folded can radically alter its properties, changing its stability and how it interacts with other substances. Some proteins act as folding chaperones, influencing the folding of other proteins so they acquire a configuration appropriate for their function. Hultgren suspected that PapD was acting as a folding chaperone for the subunits of the pilus, causing each to assume the shape that would allow it to be incorporated into the overall pilus structure.
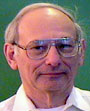
Frieden’s group captured images of PapD folding using a process they developed called stopped-flow nuclear magnetic resonance. Their results revealed that proteins that went into the pilus were folding on a strand of the PapD structure. Using other methods, they then examined whether PapD would affect the folding rate of pilus subunits.
“We showed that this isn’t just a passive process, as is true for most other chaperone proteins,” Frieden says. “PapD actually acts as a catalyst that enhances the rate of subunit folding.”
Hultgren notes that E. coli and other bacteria make many different types of pili. On its tip, each pilus type has a different sticky substance ideal for binding to a particular kind of host cell — bladder cells or kidney cells, for example.
Bacteria use different assembly processes to put together these different pili types, but many of these processes involve a PapD-like protein, according to Hultgren.
“All the members of this family of PapD-like proteins have two amino acids in a cleft structure that are key to their ability to make the pilus subunits fold correctly,” says Hultgren. “We can’t even change these amino acids in very minor ways without abolishing that function.”
In at least two ways, this inflexibility is good news for drug designers. If they can develop a drug that binds to the active site and keeps it from doing its job, that drug may be useful in treating a wide range of bacterial infections. The inflexibility also suggests bacteria will have a hard time mutating PapD in a way that abolishes a drug’s ability to bind to it while preserving the protein’s normal function.
“In this day of rising antibiotic resistance and the spread of infectious diseases, we feel it’s important to continue to investigate new and potentially better treatments for these infections,” Hultgren says.
Bann JG, Pinkner JS, Frieden C, Hultgren SJ. Catalysis of protein folding by chaperones in pathogenic bacteria. Proceedings of the National Academy of Sciences, early online edition.
Funding from the National Institutes of Health supported this research.